Infrastructure and Laboratories
Okay, let's assume we are finally on mars. Now, what will be our next step? What are we going to build? Where will we dwell? where will we carry out our research? What kind of scientific departments are we going to set up? Read to find out.
Travelling and Landing sites Infrastucture
OK now the main aspect is about the travelling and Landing sites Infrastucture which will carryout the trasportation from Earth to Mars, So we need to Build Our Martian Infrastructure so strong so that we don’t face any problem.
The energy needed for transfer between planetary orbits, or "delta-v", is lowest at intervals fixed by the synodic period. For Earth–Mars trips, this is every 26 months (2 years and 2 months), so missions are typically planned to coincide with one of these launch periods. Due to the eccentricity of Mars's orbit, the energy needed in the low-energy periods varies on roughly a 15-year cycle with the easiest periods needing only half the energy of the peaks. In the 20th century, there was a minimum in the 1969 and 1971 launch periods and another low in 1986 and 1988, then the cycle repeated. The next low-energy launch period occurs in 2033.
Several types of mission plans have been proposed, including opposition class and conjunction class or the Crocco flyby. The lowest energy transfer to Mars is a Hohmann transfer orbit, which would involve an approximately 9-month travel time from Earth to Mars, about five hundred days at Mars to wait for the transfer window to Earth, and a travel time of about 9 months to return to Earth.
Shorter Mars mission plans have round-trip flight times of 400 to 450 days, but would require significantly higher energy. A fast Mars mission of 245 days round trip could be possible with on-orbit staging. In 2014 ballistic capture was proposed, which may reduce fuel cost and provide more flexible launch windows compared to the Hohmann.
In the Crocco grand tour, a crewed spacecraft would get a flyby of Mars and Venus in under a year in space. Some flyby mission architectures can also be extended to include a style of Mars landing with a flyby excursion lander spacecraft. Proposed by R. Titus in 1966, it involved a short stay lander-ascent vehicle that would separate from a "parent" Earth-Mars transfer craft prior to its flyby of Mars. The Ascent-Descent lander would arrive sooner and either go into orbit around Mars or land, and depending on the design offer perhaps 10–30 days before it needed to launch itself back to the main transfer vehicle. (see also Mars flyby).
Aerobraking at Mars was estimated in the 1980s to cut the mass of a Mars mission lifting off from Earth by half. As a result, Mars missions have designed interplanetary spacecraft and landers capable of aero-braking.
Oxygen Plant On Mars
NASA's Perseverance rover has produced pure oxygen on Mars. The mission's MOXIE demonstration shows it's possible to convert the Red Planet's carbon dioxide-rich atmosphere into usable oxygen for astronauts. NASA's Perseverance rover has successfully generated breathable oxygen on Mars.
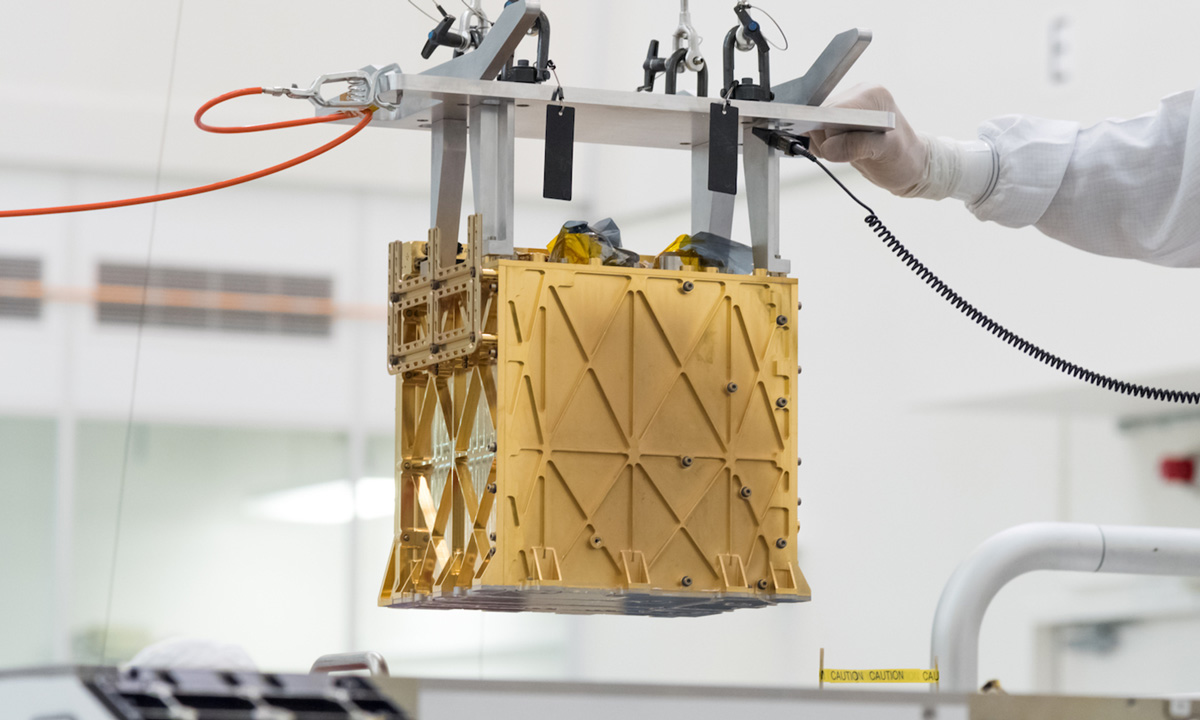
Tech Specs
Main Job | To produce oxygen from the Martian carbon-dioxide atmosphere |
Location | Inside the rover (front, right side) |
Mass | 17.1 kilograms |
Weight | 37.7 pounds on Earth, 14.14 pounds on Mars |
Power | 300 watts |
Volume | 9.4 x 9.4 x 12.2 inches (23.9 x 23.9 x 30.9 centimeters) |
Oxygen Production Rate | Up to 10 grams per hour (At least 0.022 pounds per hour) |
Operation Time | Approximately one hour of oxygen (O2) production per experiment, which will be scheduled intermittently over the duration of the mission. |
"When we send humans to Mars, we will want them to return safely, and to do that they need a rocket to lift off the planet. Liquid oxygen propellant is something we could make there and not have to bring with us. One idea would be to bring an empty oxygen tank and fill it up on Mars."
So the production of oxygen is possible on mars using suitable Infrastructure.
Here are demo oxygen plant simulation which will helpfull on mars to produce breatheable oxygen
Energy resources Infrastructure
Introduction
With the recent advances in commercial spaceflight comes a renewed public interest in colonizing Mars. However, there are significant challenges in establishing a self-sufficient outpost on the Martian surface. A principal requirement is a reliable source of energy - to keep electronics and human occupants warm in the frigid Martian weather, to enable scientific research, to produce propellant from in situ resources for return flights, and so on. To date, the two energy sources utilized for Mars missions have been sunlight and radioactive decay. A manned mission or permanent settlement would likely also have to choose between these two.Solar
Though a number of robotic probes sent to the explore the Martian surface have successfully utilized solar arrays for their power needs, such an approach would have trouble scaling to support human habitation. The principal concern with using solar power to support a mission is intermittency: solar panels only provide power when there is sunlight. This is a familiar problem on Earth, and a major obstacle to wider integration of renewables into the grid. The intermittency problem on Mars is more pernicious: enormous global dust storms envelop the planet typically once a year from 35 to 70 or more Martian days (sols). These dust storms tend to have an opacity, or optical depth, of at least 1 - meaning that the solar flux at the top of the atmosphere is attenuated to less than e-1 = 0.37 (37%) of its original value when it reaches the surface. In addition, because Mars is farther from the Sun than Earth is, it already only receives roughly half the average solar irradiance. This intermittency introduced by multi-month dust storms, combined with the usual diurnal oscillation in solar flux, would necessitate a considerable amount of energy storage.Nuclear
Nuclear power is an attractive alternative to solar for several reasons. Its power output is constant in time, meaning less risk of prolonged power shortages that could prove hazardous to a human crew. It also weighs less per nameplate capacity than does solar when considering a Mars operating environment - a 2016 NASA study found that about 18,000 kg of solar power generation equipment would be needed to match the output of a 9000 kg fission system. This was considering a relatively small system meant to provide 21 kW peak electric power for a handful of astronauts, which translates to roughly 1.2 watts per kilogram for the solar power system and 2.3 watts per kilogram for the nuclear system.The nighttime temperature on Mars as measured by the Opportunity rover reach as low as -98°C with diurnal temperature variations of up to 100°C, so even a temporary power loss in such an environment could quickly become life-threatening as the heating systems fail.This presents another advantage of nuclear power: even in the event of an electrical fault, the passive heat from the reactor or radioisotopes could be used to warm the habitat.
A nuclear power system on Mars would look quite different from a fission nuclear plant on Earth. It would be small, modular, and self-contained, and rather than using a large steam turbine it would likely use a thermoelectric generator to convert heat from non-fissile radioisotopes, most commonly Pu-238, directly into electricity. Radioisotope thermoelectric generators (RTGs) have already been proven effective in multiple space missions, including the Pioneer and Voyager spacecraft as well as the more recent Mars Science Laboratory (Curiosity) rover. However, NASA is also currently evaluating the performance of its prototype "Kilopower" system, which would use a fissile uranium core instead of plutonium, and a Stirling engine instead of an RTG, to provide 1-10 kW of power per reactor. Though still unproven, this design would be more scalable because of the greater availability of uranium fuel, and would enable higher-powered systems than previously available with RTGs.However, because fissile radioisotopes have the inherent danger of a meltdown, the RTG design may yet win out due to safety considerations.Government
I have written before of the challenges of governing an extraterrestrial colony. The early missions – particularly those involving space agencies – will almost certainly be run with a hierarchical command system. The past 50 years of human spaceflight have taught us that, in the extreme environment of space, this is the safest way. However, there is a fine line between a Star Trek-type command structure and a brutal military dictatorship, and as the settlement matures, some sort of democracy is going to be favoured.
“A space colony is a tyranny-prone environment,” says Charles Cockell, an astrobiologist from the University of Edinburgh who is also leading research on developing a constitution for space habitats. “If somebody gets control of oxygen, they could very well have control over the whole population and threaten dire consequences in return for extraordinary levels of power.”
As a commander of a space colony on Earth, Tarvin is one of the few people to have any experience of overseeing a Mars base. “It’s certainly not a Star Trek-style military environment,” he says. “It’s a small group of highly motivated people and it really doesn’t take much effort to manage them.” A government also needs all the structures that go with it. Any new society needs an economy as well as systems to maintain the habitat, provide employment, health, childcare, social care and education. In short: Mars needs bureaucrats.
Human well Beings Infrastructure
We are going to Mars means Safety, Needs, Medical treatment, Foods availability, Water Plant, etc all are important aspect to be manege on mars. So that we can research more and explore the Mars safely.
In that sense we need to build our Infrastructure more strong and safe
Water plant
Almost all water on Mars today exists as ice, though it also exists in small quantities as vapor in the atmosphere.What was thought to be low-volume liquid brines in shallow Martian soil, also called recurrent slope lineae, may be grains of flowing sand and dust slipping downhill to make dark streaks. The only place where water ice is visible at the surface is at the north polar ice cap. Abundant water ice is also present beneath the permanent carbon dioxide ice cap at the Martian south pole and in the shallow subsurface at more temperate conditions. More than 5 million km3 of ice have been detected at or near the surface of Mars, enough to cover the whole planet to a depth of 35 meters (115 ft). Even more ice is likely to be locked away in the deep subsurface. Some liquid water may occur transiently on the Martian surface today, but limited to traces of dissolved moisture from the atmosphere and thin films, which are challenging environments for known life.No large standing bodies of liquid water exist on the planet's surface, because the atmospheric pressure there averages just 610 pascals (0.088 psi), a figure slightly below the vapor pressure of water at its triple point; under average Martian conditions, warming water on the Martian surface would sublime meaning transition directly from solid to vapor; conversely, cooling water would deposit meaning transition directly from vapor to solid. Before about 3.8 billion years ago, Mars may have had a denser atmosphere and higher surface temperatures, allowing vast amounts of liquid water on the surface,possibly including a large ocean that may have covered one-third of the planet. Water has also apparently flowed across the surface for short periods at various intervals more recently in Mars' history. Aeolis Palus in Gale Crater, explored by the Curiosity rover, is the geological remains of an ancient freshwater lake that could have been a hospitable environment for microbial life
On Mars, water can be extracted from the soil. The rover will select the location for the settlement primarily based on the water content in the soil. We expect this to be at a latitude of between 40 and 45 degrees North latitude. Water extraction will be performed by the life support units. The rover will deposit soil into a water extractor in the life support units. The water extractor will heat the soil until the water evaporates. The evaporated water will be condensed and stored, the dry soil expelled, and the process repeated to extract more water.
About 1500 liters of reserve water will be stored in each life support unit, which will be consumed primarily at night, and during periods of protracted low power availability, for example during dust storms.
Since Mars has gravity, water can be used in the same way as on Earth. Each astronaut will be able to use about 50 liters of water per day. The water will be recycled, which takes much less energy than extracting it from the Martian soil. Only water that can not be recycled will be replaced by water extracted from the soil.Laboratories
What Will a Lab on Mars Be Like?
A lab on Mars would be essential to future manned missions and the search for life.
Currently, the only analytical lab on Mars is a suite of instruments wheeling across the planet’s surface on the Curiosity rover, managed by the Mars Science Laboratory mission. Over the four years it’s been on the Red Planet, Curiosity’s mission has been to analyze soil and rock samples to help determine whether microbes ever lived there. “While Curiosity and previous rovers have revolutionized our understanding of Mars, we are really at the point we are ready for human explorers/ scientists, to take our knowledge of Mars, a whole new world, to the next level,” says Richard Davis, the assistant director for science and exploration in the Planetary Science Division of the Science Mission Directorate at NASA headquarters (Washington, D.C.).
“It may be that we will not be able to definitively answer the question of whether life developed on Mars until we have adaptable, creative, [and] intuitive humans on the surface of Mars looking for signs of life,” he says. Such missions would require a semi-permanent laboratory on Mars, and while NASA hasn’t yet revealed exactly what that lab would look like, Davis says it will probably be similar in size and appearance to the lab module onboard the International Space Station, with up to four researchers able to work at the same time. As far as exact location, he adds that in October 2015, NASA started the selection process for the site of a human base/research station on Mars, with 47 possible landing sites in the running.